This post is part 3 of my special report on the #ASP2012 (Australia) meeting at Launceston, see part 1 here and part 2 here.
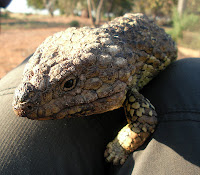 |
Photo by Caroline Wohlfeil |
There were a number of interesting talks from the wildlife session, first up was a talk by Caroline Wohlfeil - a student from
Michael Bull's lab. She gave a talk on
sleepy lizards (see right) and the reptile tick,
Bothriocroton hydrosauri. These ticks go through 3 stages in their life-cycle, alternating between feeding on a lizard and dropping off in a sheltered area to develop once they are fully engorged. It is in this latter stage that there ticks are transmitted - when lizards take shelter at refuges that have previously been used by infected lizards, they pick up ticks that were dropped off from the previous lizard. Using GPS loggers which continuously recorded the lizard's activity and location, Caroline was able to use that data to work out how often each of the tracked lizard had opportunities for infection. Her network analysis revealed that lizards that are highly-connected also had higher tick loads.
This was followed with a talk by Luz Botero Gomez, a student at Murdoch University, on trypanosome infections in little marsupial call the Brushed-Tail Bettong or
Woylie. We have previously covered trypanosomes in another marsupials on this blog, namely the
koala, but as it turns out, there is a great diversity of
Trypanosoma in native marsupials - most of it still unknown. Woylie are known to be infected with 3 species -
T. cruzi (the species which causes
Chagas disease),
T. copemani, and an as yet unnamed clade of
Trypanosoma. Some of those
Trypanosoma species are also found in other Australian marsupials but only the woylie is known to carry all three. Much like the
koala-infecting trypanosome,
T. copemani seems to only cause problem when it occurs in mixed infection with other
Trypanosoma species - such co-infections can leads to inflammations and lesions in the tissue. In addition, these different trypanosomes also seem to have varying degrees of tissue specificity, with some species occurring in the blood, while other in muscle tissues, but overall mixed infections are more likely to occur in organs and muscles. Given the Woylie is currently critically endangered, it is very important to know what kind of diseases are induced by these trypanosomes and how it is affected by whether they are single or mixed infections.
For a change of pace from parasites threatening a critically endangered mammal, Amanda Ash (also from Murdoch University) presented a talk call "Parasite: embrace not erase" which praised the important functional roles played by parasites in various ecosystems, and discussed the results of a study she conducted looking at the inestinal parasites of
African Painted Dogs. She collected fecal samples from captive and wild Painted Dogs and compared the types of parasite eggs and cysts found in those sample. She found that the intestinal fauna of captive dogs was comparatively depauperated, populated only by
Giardia whereas the wild dogs had a more eclectic mix of tapeworms, hookworms, and various protozoan parasites - in addition
Giardia. Another stark contrast between the captive and wild dogs is that whereas parasitic infection was ubiquitous in the wild population, with 99% had some sort of parasite, only 15% of the captive dogs carried intestinal parasites of some sort.
This has enormous implications for conservation measures such as captive breeding programs - animals which have not been exposed to a wide range of parasites and pathogens can grow up to become immunologically naive so that when they are release into the wild, they may not be able to cope with the wide range of parasites they encounter. In addition, it is unknown what other physiological side-effects may result from lack of exposure to parasites. According to the
hygiene hypothesis, the numerous types of allergies and auto-immune diseases which afflict some of us living in western societies have result from the lack of exposure to
parasitic worms which are masters at
manipulating and
modulating our immune system. By limiting both the prevalence and variety of parasitic infections in those captive African Painted Dogs, are we consigning them to the same fate?
During the poster presentation, we saw some students who have come up with creative ways of presenting a 2 min talk - a student from James Cook University read a poem about whether wild dingoes pose a threat to the health of Indigenous communities in Queensland, while a student from University of Western Australia was
literally singing the praises of using volatile chemicals for malaria parasite detection. Some of the most fascinating poster talks may present nightmarish scenarios to some people, but for different reasons.
For non-parasitologists, the
tongue-biter seems like a one-off freak of nature. But in fact there are actually many species of tongue-biting isopods and
other parasitic crustaceans which inhabit the mouth, gills, and branchial cavity of fish. One genus of tongue-biter isopod -
Ceratothoa - encompasses 29 known species worldwide, 6 of which are found in Australia waters. However, a new study by Melissa Martin from University of Tasmania revealed that there at least 12 species of
Ceratothoa (from 7 families of fish), and 4 of them are new to science.
Dinh Hoai Truong, a student from the
Hutson lab at James Cook University presented a horror of a different kind - less visceral than having a parasite in your mouth, but more of a biosecurity nightmare to aquaculturists. He presented a poster on
Neobenedenia - a hermaphroditic
monogenean which infects the skin of
Barramundi. His experiment showed that a single
Neobenedenia is able produce eggs through self-fertilisation for consecutive generations without suffering any deleterious effects of inbreeding - each consecutive generation of inbred
Neobenedenia are just as infective as the last. This means that even a single worm can start an entire sustained infestation at a fish farm. Unlike the widespread monogenean
Gyrodactylus - a notorious aquacuture pest which has the viviparous "Russian Doll"-style "worm-within-a-worm" reproductive set-up (which allows them to swarm a fish like aphids on a rose bush) -
Neobenedenia does what most monogeneans do and simply produce eggs. However because they are able to self-fertilise and have very short generation time, they can still become a serious pest to aquaculture.
In the next and final post on #ASP2012 (Australia), we will talk about how environmental factors can affect the generation time of
Neobenedenia, and meet many other weird and wonderful marine parasites.
Next post: Swimming with the Parasites