Through out 2011, we have been featuring more weird and wonderful parasites as we have done in 2010, but on top of that we have also been featuring some of the latest pieces of research being published on all manners of parasitic and infectious organisms which may not have been covered elsewhere in the blogosphere.
Just this year we saw a molecular study that revealed the transmission pathway of a great white shark tapeworm via dolphin blubber, a koala blood parasite named after Steve Irwin, a nematode which infiltrate pine trees via borrowed genes from fungi, a parasitic plant which disperse its seeds via beetles, and a little digenean fluke which changed the face of an entire mudflat, just to name a handful out of dozens that we have featured.
So in 2011, we have gone beyond merely showing you the parasites, but also told you about the research which are being conducted behind the scene to work out how they live. At the same time, you also got a bit of insight into the scientific process, and how knowledge accumulate and grow over time.
In 2012, we will continue to bring you new and exciting research on parasites and parasitism - publications which we found interesting but might not have received their share of fanfare and press releases (as I type this up, I have at least another 4 paper waiting in line to write up. And no doubt I'll find another 4 to write about by the time I'm done with those).
Of course, research on a particular host-parasite system does not simply enter suspended animation after a single study has been published. For many lab groups, the parasite we have featured on this blog form the basis of their scientific research, and the newly published paper we have chosen to feature here merely represents a thin cross-section of their ongoing research program. So in 2012 we might also be revisiting some of the parasites that we have previously featured on the blog, and fill you in on the latest updates as they hit the press.
Of course, you can now also find us on other forms of social media where we will be posting about updates to the blog. Susan is on Twitter , and you can find myself on Google Plus.
Here's to another year of parasitism - the most common way of life on this planet!
"So, naturalists observe, a flea has smaller fleas that on him prey; and these have smaller still to bite ’em; and so proceed ad infinitum."
- Jonathan Swift
December 31, 2011
December 17, 2011
Hematodinium sp.
Hematodinium and related species are dinoflagellates, and while most dinoflagellate are free-living, this species belongs to a group which have evolved to be parasites, with many different species infecting a wide variety of hosts. While several different stages of the parasite have been isolated from the blood of infected crabs, little is known about how they are transmitted between hosts, nor the inner life of those different stages in the hosts. Because many parasites live enclosed within the body of their hosts, it is almost impossible to directly observe how they live and grow the way you might be able to observe a fish or a bird. Ideally, if you can isolate a parasite out of its host, put in it a clear container which closely mimics the conditions found within its host, and still have it complete its life-cycle, then you can find out a lot more about how it lives.
Recently, a group of researchers from Virginia were able to successfully complete the life-cycle of Hematodinium - in vitro - which means they were able to grow it in a culture of chemical broth that sustained the parasite's every need, without any host animals involved. This was accomplished through a painstaking series of transfers, starting with isolating the parasite from infected crabs, then moving each stage into different culture mixes as it grew, all while keeping the conditions as sterile as possible. Out of the 10 isolates they attempted to grow, only 4 successfully completed their life-cycle in vitro. The researchers also found out that the parasite grows best in the dark, and indeed light exposure kills them within weeks, which makes sense given that it is pretty dark inside a crab (a variation on the Marx Brother joke).
Through this in vitro technique, they were able observe the different parasitic stages of Hematodinium directly, and view them as they would have been while floating in the blood and organs of a blue crab. They noted that when Hematodinium cells first enter the crab as "dinospores," they turn into a worm-shaped form called a "filamentous trophont" (see the accompanying photo which was from a figure in the paper). About a month after that, the cells begin transforming into clumps that are composed of multiple clones of the original infection stage. These clumps then grow into a stage called an "arachnoid trophont," which resembles a blob with numerous tendrils around its fringe (which would be embedded in the hepatopancreas of the crab). These clumps tend to merge and form larger blobs as they come into contact with each other. When those "arachnoid trophonts" fully develop, the cells in the middle of the blob start producing spores that eventually turn into the infective dinospores that escape from the crab to infect new hosts, starting the life-cycle anew.
Reference:
Li, C., Miller, T.L., Small, H.J. and Shields, J.D. (2011) In vitro culture and developmental cycle of the parasitic dinoflagellate Hematodinium sp. from the blue crab Callinectes sapidus. Parasitology 138:1924-1934.
Postscript: Three days after this post went up, I was contacted by Peter Coffey, who used to work on this species of parasite with a bit of additional information/correction: I just have one quick comment on the first sentence in your post. In blue crabs we don't see the same bitter flavor that we do in Alaskan Tanner and Snow Crabs, so we haven't been calling infections in blue crabs BCD.
Thanks Peter!
December 8, 2011
Lepeophtheirus acutus
Today, we are featuring a paper which reported on a grey reef shark (Carcharhinus amblyrhynchos) at Burger's Zoo in the Netherlands that had to be euthanized. "Wait a sec!" you think, "Isn't this supposed to be a blog about parasites? I didn't come here for dead sharks!" Well, just calm down before you close your browser tab in outrage. This particular shark actually succumbed due to a heavy infection of today's parasite - Lepeophtheirus acutus. This parasite is in the same genus as other fish lice that we have previously featured on this blog, but very little is known about this particular species. Prior to this incident, it has only been reported once from the wild, and it was found on the back of a ribbon-tailed stingray (Taeniura lymma), not a shark and certainly nothing was known about how harmful it can be to its host.
From what the staff at the aquarium could work out, this deadly little crustacean was introduced to the facility by an infected male zebra shark (Stegostoma fasciatum) collected off Cairns, Australia on the Great Barrier Reef, which appeared perfectly healthy at the time and passed quarantine. However, about 2 weeks after he was introduced into the aquaria with the other fishes, he started acting weird. At the same time, the grey reef shark mentioned at the start of this post became lethargic and ceased to eat regularly, and about a month after that, both sharks were afflicted with swollen and opaque eyes. Despite the best efforts of the staff to put the infected sharks in quarantine, filter the water with activated carbon, and give them anti-parasite drugs, they were unable to save the grey reef shark, by which time it was swimming with its mouth wide open, not eating at all, and its eyes had deteriorated even further, so the decision was made to euthanize the long-suffering shark.
A necropsy revealed the identity of the killer - a parasitic copepod - most of which were found around the shark's eyes which caused them to become swollen and covered in mucus, and the mouth which led to bleeding gums. The parasite was also found on a female zebra shark and a shovelnose ray (Glaucostegus typus) which shared the aquaria with the deceased grey reef shark. Notably, the blacktip reef shark (Carcharhinus melanopterus) and blacktip sharks (Carcharhinus limbatus) which swam in the same water alongside those infected sharks did not become infected, nor did the many different species bony fishes sharing the same tanks and water. This indicates that L. acutus does display some selectivity in the type of host it infects, with a particular preference for elasmobranchs (sharks and rays), and even then only certain species within that group.
Other than the dead grey reef shark, the other infected sharks survived and recovered fully after treatment. However, this incident shows how outbreaks of infectious diseases can be a big problem for animals in the confined conditions of captivity. In the case of L. acutus, its small size, semi-transparent body, its tendency to infect parts of the host that are difficult to inspect (for example, inside the mouth), and the fact that nothing is know about its ecology meant that the staff had not anticipated such an outbreak. It was the first documented case of infection by a parasitic copepod that led to a shark dying in captivity. This case also illustrates the importance of thorough quarantine procedures, especially when introducing new animals into any facility, as captive conditions can seriously alter the transmission dynamics and pathology of relatively harmless parasites.
Image from figure in the paper.
Reference:
Kik, M.J.L., Janse, M., Benz, G.W. (2011) The sea louse Lepeophtheirus acutus (Caligidae, Siphonostomatoida, Copepoda) as a pathogen of aquarium-held elasmobranchs. Journal of Fish Diseases 34: 793-799,
December 1, 2011
Rhinanthus minor
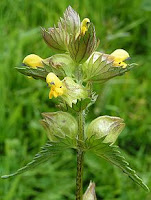
Many parasites can have substantial effects on their hosts, but their impact can often extend to other organisms in the environment. Today's parasite is one of the more pretty-looking ones which we have featured in a while - as opposed to the usual worms and lice, today we are featuring a flowering plant - the yellow rattle. But don't let its pretty yellow flowers fool you, Rhinanthus minor is a ruthless parasite.
It is a hemiparasite (like the mistletoe , which becomes rather popular during this time of the year). The plant overwinters as seed in the soil and germinates during spring, penetrating into the roots of its host plants where it can suck out nutrients and water from the plant's xylem tissue. The yellow rattle is a fast growing plant - it flowers 12 weeks after germination and 3 weeks after that it produces seeds that are loosely held in dry capsules, which gives the plant its name. The yellow rattle often share its host plants with a range of insects, so a group of researchers in the UK decided to look at how this hemiparasite can affect those insects. Specifically, they looked at the effects of R. minor on insects that exploit plants in different ways; the aphid that feeds on the sugary sap flowing in the plant's phloem, the spittle bug, which taps into the xylem that transports water and other nutrients, and the grasshopper, which simply chews on leaves.
The researchers predicted that over the course of its growth, the yellow rattle would affect those insects differently. They were expecting that it would negatively impact on the spittle bug, because that insect and the hemiparasite both draw their nutrients from the host's xylem. But as is often the case in science, they found something unexpected.
First of all, they found that the effect R. minor had on those insects depended upon the parasite's growth stage, and it becomes most pronounced when the yellow rattle reaches its peak biomass and begins setting seeds. However in contrast to what they were expecting, spittle bugs actually preferred plants parasitised by R. minor. But the insect that benefited the most from the hemiparasite's presence were the aphids. Not only did they prefer sharing a host plant with the yellow rattle (there were three times as many aphids on plants with R. minor compared to uninfected plants), they also tend to breed more on infected plants. What about the grasshoppers? Grasshoppers were not all that affected by the presence of the yellow rattle either way.
The mechanism behind why the yellow rattle makes its host more attractive to plant-feeding insects is currently unknown. However, it may have something to do with the hemiparasite altering the water content of the host plant, or changing the composition of the phloem sap, which makes it more nutritious to aphids. Either way, it seems that at least for some insects, sharing a plant with a hemiparasite might actually be a good thing.
Image from the Wikipedia.
Reference:
Ewald, N.C., John, E.A. and Hartley, S. (2011) Responses of insect herbivores to sharing a host plant with a hemiparasite: impacts on preference and performance differe with feeding guild. Ecological Entomology 36: 596-604.
November 22, 2011
Ophiocordyceps unilateralis
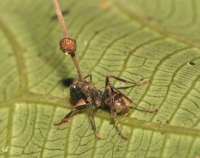
Have you ever been so intoxicated that you start walking erratically, stumble away from your friends, stagger around in circles, clamber onto things that you wouldn't normally be seen near, and the next thing you know, you are strapped down in unfamiliar surroundings, unable to extricate yourself? Well, that pretty much describe what happens to ants which become infected with the famous "zombie ant" fungus - Ophiocordyceps unilateralis.
Much has been written about this famous fungus which turns ants into zombies - it is a parasite which captures the same part of our psyche as the monstrosities of horror movies, and there is evidence to suggest that these fungi have been tormenting ants for at least tens of millions of years. But despite all that attention, few people have actually witnessed or documented the sequence of behaviour leading up to the infected ant's paralysis and death. But in a paper published this year, a group of researchers followed the behaviour of ants infected with the famous "zombie"-inducing fungus and compare them to their uninfected brethren.
They noticed a few peculiarities with the behavioural repertoire of infected ants which stood out. While healthy ants studiously stick to the usual lanes of ant traffic, climbing into the canopy to forage with all the other busy worker ants, "zombie ants" are loners which meander around in the understory by themselves, are unresponsive to most stimuli, and frequently stumble and fall from the branches they are walking on. Essentially, the ants act absolutely drunk, indeed, the researchers described the behaviour of the "zombie ants" as a "drunkard's walk" in their paper. Another weird thing that infected ants start doing is their tendency to crawl all over and bite into leaves - something which healthy ants don't tend to do. There's a good reason why the fungus steers the ant towards leaves and afflict it with this oral fixation - it is preparing it for the next step in the fungus' development.
For the fungus to successfully reproduce, the ant must die - but it must die in a particular position to maximise the viability and dispersal of the fungal spores, specifically in the humid understory, hanging from the underside of a leaf, about 25 cm (about 10 inches) above the ground. But once the fungus maneuver the ant into position, how does it get the host to comply and stay there? The researchers made fine histological cross-section of the infected ant's head and found that once the fungus has made the ant locks its mandible in place, it busily gets to work dissolving the muscles which control those mandibles, ensuring that the ant will be locked in a death grip forevermore. A few days after the ant dies while gripping onto, the fungal stalk emerges from the head of the ant, ready to spray its spores down to the soil below to create more drunken "zombie ants".
Image from the Wikipedia.
Postscript: A few hours after I wrote this post, I found out that Carl Zimmer has already written about this study (why, of course! *facepalm*), so if you want to read his version instead, you can see it here.
November 11, 2011
Polypocephalus sp.
Source: Carreon, N., Z. Faulkes, and B. L. Fredensborg. 2011. Polypocephalus sp. infects the nervous system and increases activity of commercially harvested shrimp. Journal of Parasitology 97:755-759
Image from figure of that paper.
November 3, 2011
Bursaphelenchus xylophilus
Today's parasite is the nematode Bursaphelenchus xylophilus, a well-known tree-killer responsible for the devastating plant disease known as pine wilt. Originating in North America, it has since been spread over much of Asia, and has recently been introduced to Europe. This nematode is transported by longhorn beetles known as "pine sawyers", and gain initial access to the tree through the feeding wound created by that insect. So the arrival of a B. xylophilus-laden beetle pretty much amounts to a death sentence for a pine tree. While pine trees in North America have coevolved with B. xylophilus and developed resistance or tolerance for the parasite, it has caused widespread wilting and death to the pine trees of Japan. So how can such a tiny worm bring down an entire pine forest?
For B. xylophilus, or any other plant parasites for that matter, a tree is a formidable fortress - protected by walls and scaffolding of tough cellulose, and canals of deadly resin. Plant cell wall presents the main barrier to any plant parasites - it is a tough material to break down, and most animals are incapable of doing so without the aid of symbiotic microbes. In addition, the vascular tissue of many coniferous plants like pine are saturated with resin - a thick, sticky cocktail of aromatic chemicals (from which we derive many useful substances including solvents, varnishes, adhesive and perfume) which would overwhelm and kill most invaders. Yet none of those defenses seem to deter B. xylophilus - not only can it break through the thick cellulose barrier of the pine tree, it actually lives within the resin canals of its host, which is practically the most lethal place within the tree. It would be akin to living in a moat of toxic tar.
A recent study published in PLoS Pathogens on the genome of B. xylophilus offers vital clues to how this nematode exploits its pine tree host. One of the most important enzymes for plant exploitation is cellulase - it is used to break down cellulose structures and allow potential parasites to enter and navigate through the host. Bursaphelenchus xylophilus is able to produce a unique combination of 34 enzymes for breaking down cellulose and carries a diverse suite of genes for producing enzymes that detoxify the aromatic compounds found in resin. So how did this tree killer acquire the necessary molecular machinery to invade and disarm its host?
The wide range of detoxifying genes in the B. xylophilus genome appear to be multiple duplication of pre-existing genes which are also found in other nematodes, such as the well-known standard lab worm Caenorhabditis elegans - B. xylophilus just happen to have more of copies of those genes to cope with the wider array of toxins it encounters. However, the cellulase genes have a much more unusual origin. Out of the 34 cellulase enzymes produced by B. xylophilus, 11 of those enzymes are not found in any other nematode, but are most similar to those produced by fungi. So how does a nematode end up producing fungal enzymes?
The answer might be through horizontal gene transfer (HGT). The closest living relatives of B. xylophilus are fungi-eating worms which are transported by beetles to dead and dying trees. Once they reach their destination, they disembark from their beetle vectors and feed on the fungi which have colonised the dead trees. In a case of you are what you eat, the ancestors of B. xylophilus appeared to have incorporated a whole suite of useful genes from their food, allowing them to bypass the process of feeding on fungi which are growing on dead trees and just go straight to breaking down live plant tissue.
Image from figure of the paper.
Reference:
October 23, 2011
Nicothoë astaci
The parasite we are featuring today is Nicothoë astaci, the "lobster louse." Despite its name, it is not a "louse" (true lice are insects) as such, but rather a copepod (a type of crustacean), just like the salmon lice we have previously featured on this blog. But whereas salmon lice are well-studied due to their economic impact on salmonid fisheries (especially on farmed fishes), far less is know about the lobster louse. Despite having been recorded on the European lobster (Homarus gammarus) since the 1950s, to this day there is very little known about this parasite, including the type of pathology it causes, its complete life-cycle, or even what the male of the species looks like (parasitic copepods often have cryptic or dwarf males which are very elusive).
The paper we are looking at today is taking the first step to rectifying that situation. The photo (from the paper itself) depicts larval stages of N. astraci, with the arrows indicating the oral cone,the structure this parasite uses (along with its front pairs of legs) to attach itself to the host's gill filament and feed on its blood. While the larval stage looks like a rather ordinary copepod, as it matures into an adult, it morphs into what looks like a miniature boomerang with a pair of stretched out "wings" on either side, and a pair of bulbous egg sacs dangling from its rear end. The attachment and feeding activity of the lobster louse can cause pronounced physical damage to the lobster's gill filaments.
As with any kind of infection, you would expect to see some kind of cellular response. While the innate immune systems of invertebrates like lobsters are not as sophisticated as the adaptive immune system of vertebrate animals such as ourselves, they can present a formidable challenge to any would-be intruder (to see an example of what the cellular defence of a crustacean can do to a parasite, click here). Basically, the crustacean's equivalent of blood cells wrap themselves around the parasite or pathogen and initiate the process of melanization, where the intruder becomes entombed in a hardened capsule of melanin (the pigment which determines our skin colour). The researcher did find signs of melanization and other cellular disruption throughout the gills of infected lobsters, but none of it was near the lobster louse's attachment point.
So the lobster's immune system recognizes the presence of an intruder, but is unable to pinpoint and focus its wrath on the parasite. The authors of this paper suggest that this indicates the lobster louse is able to somehow interfere with the lobster's defensive mechanism so that it can blood-feed in peace. The mechanism through which the lobster louse disrupts this particular aspect of host physiology is yet to be uncovered, along with much of the parasite's ecology and life-cycle. Hopefully, with further research on this host-parasite system, this situation will change in the future.
Image from the paper.
Reference:
Wootton EC, Pope EC, Vogan CL, Roberts EC, Davies CE, Rowley AF. (2011) Morphology and pathology of the ectoparasitic copepod, Nicothoë astaci ('lobster louse') in the European lobster, Homarus gammarus. Parasitology 138:1285-1295.
October 12, 2011
Maritrema subdolum
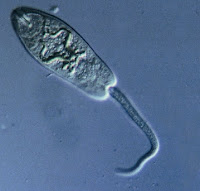
This is a story about two little crustaceans and a parasitic fluke. Corophium volutator and Corophium arenarium are amphipods that make a living tunneling in the mudflats on the coast of the Danish Wadden Sea. They are also host to many species of parasitic flukes, and one of them is the parasite we are featuring today - Maritrema subdolum. Last year, we featured a related species from New Zealand - M. novaezealandensis - and much like its Kiwi cousin, M. subdolum is the bane of the local crustacean population. However, M. subdolum does not affect all of its crustacean hosts equally and this has some important ecological consequences.
Out on the Danish mudflats, C. volutator is king. Of the two Corophium species, it is the stronger competitor, reaching much higher abundances and generally making life difficult for C. arenarium. But along comes M. subdolum, which evens out the playing field. Living alongside those little crustaceans are mud snails, and during early spring they can become extremely abundant, with over 25000 snails per square metre. By summer, almost half of those snails are infected with M. subdolum, which turn them into little parasite factories, each cloning a massive reserve of parasite larval stages call cercariae that are then unleashed into the environment to infect the amphipods. The main trigger for releasing these cercariae is high temperature, and during 1990 there was a bout of unusually high temperature during summer that has been linked to North Atlantic Oscillation (NAO), a phenomenon that plays a major role in determining climatic conditions in the northern hemisphere.
All of this combined into a perfect storm that devastated the C. volutator population. Triggered by high temperature, all the infected snails released their payload of parasites into the surrounding waters. Each infected snail can release hundreds of cercariae, and with thousands of snails per square metre, the shallow waters of the mudflats turned into a seething parasite soup. To the amphipod, this amounted to being in a shooting range, as each cercaria is armed with glands of digestive enzymes and a scalpel-like organ call a stylet with that they use to puncture the amphipod's exoskeleton. For many C. volutator, the outcome of being attacked by a swarm of these little horrors was terminal, and this resulted in a dramatic decline in their population. However, for some reason we still don't know, the C. arenarium population was able to weather the M. subdolum storm unscathed, either because they tolerated the parasite swarm, or because they were simply not the preferred target. Either way, with the collapse of the C. volutator population, in the next season, C. arenarium was able to succeed them and become the dominant amphipod species on the Danish mudflat.
And this dramatic ecological change was ultimately brought about by a little parasite.
Photo by Kim Mouritsen
Reference:
Larsen, M.H., Jensen, K.T. and Mouritsen, K.M. (2011) Climate influences parasite-mediated competitive release. Parasitology 138: 1436-1441
October 3, 2011
Metarhizium acridum
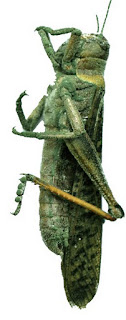
So why is M. acridum so picky while its close relative is so indiscriminate? Amazingly, it appears to come down to a single gene call Mest1 - a gene present in M. robertsii, but is absent in M. acridum. To find out the function of this gene, a group of researchers in China created a mutant M. robertsii strain which has a non-functioning copy of Mest1. This mutant lost its ability to infect most insects - except grasshoppers and locusts - which happens to be the speciality of M. acridium. In parallel, the researchers also inserted functional copies of Mest1 into M. acridum. The insertion of this single gene allowed M. acridium to infect a wider range of insects.
What is so special about Mest1? In M. robertsii, Mest1 is expressed during spore germination, and plays an important role in initiating the infection process. Mest1 expression can be triggered by a range of stimuli including nutrient poor conditions or contact with insect cuticle. Metarhizium acridium has other genes playing the role of Mest1, but they are triggered by substances which are present only in the waxy coating of grasshoppers and locusts. So if its spores land on other insects such as caterpillars which have a different type of coating, M. acridum fails to germinate because the appropriate stimuli are absent. Thus, the insertion of Mest1 into M. acridium allows the fungus to bypass those usual stimuli and begin germinating under a wider range of conditions
Host specificity is one of the central question in the evolutionary biology of parasitic organisms. In this case, we can see how a single gene can changed this otherwise specialist pathogen into a broad-spectrum generalist.
September 26, 2011
Parvilucifera sinerae
For P. sinerae, infecting its host is not an easy task - not only does it have to find a swarm of its tiny host in the vast ocean, it also needs to make contact and accomplish what amounts to a cellular heist - the parasite needs to break through the protective shell of the alga in order to steal its valuable content. As you can imagine, during such an intense operation, being jostled around will probably throw you off your game. And indeed that is what a group of scientists in Spain have found. It appears that even a slight turbulence is enough to reduce the infection success of P. sinerae and that it performs best under calm, still conditions. These researchers suggested that turbulence would erode the zone of chemical emission around the dinoflagellate, making them more difficult to detect. Turbulence would also shorten the period of time which P. sinerae are in constant contact with the host cell - which is a necessary precondition for the parasite to perform its little cellular heist.
While both P. sinerae and its host are tiny, their interactions can have far-reaching ecological consequences, and as explained earlier they are among the most important organisms on the planet. In addition, parasitic killers, such as today's parasite, have been suggested as a possible biological control for harmful algal blooms, but it is like that the effectiveness of any such control would be at the mercy of environmental factors such as small-scale marine turbulence.
Image from figure of the paper.
Reference:
Llaveria, G., Garcés, E., Ross, O.N., Figueroa, R.I., Sampedro, N. and Berdalet, E. (2010) Small-scale turbulence can reduce parasite infectivity to dinoflagellates. Marine Ecology Progress Series 412: 45-56.
September 16, 2011
Sphaeromyxa cannolii
Image from the paper.
Reference: Sears, B.F., P. Anderson, and E.C. Greiner. 2011. A new species of myxosporean (Sphaeromyxidae), a parasite of lined seahorses, Hippocampus erectus, from the Gulf of Mexico. Journal of Parasitology 97:713-716.
Reference: Sears, B.F., P. Anderson, and E.C. Greiner. 2011. A new species of myxosporean (Sphaeromyxidae), a parasite of lined seahorses, Hippocampus erectus, from the Gulf of Mexico. Journal of Parasitology 97:713-716.
September 12, 2011
Cardicola forsteri
Being a trematode, Cardicola fosteri must have an invertebrate host that is the source infection for the tuna. In their search for the first host of C. forsteri, researchers undertook a a truly heroic effort - sampling over 9000 (!) invertebrates, including all kinds of bivalves, snails, and polychaete worms from the pontoons on the tuna ranch and nearby areas, then meticulously dissected and examined every single one of them for parasitic infections. Those who have been following this blog would know that trematodes usually have a mollusc host in which they undergo asexual multiplication - usually a snail, but C. forsteri is very unusual - it turns out that it uses a polychaete worm, specifically tube-dwelling terebellids - also known as spaghetti worms - for asexual multiplication. Infected worms were packed with hundreds of sac-like sporocysts which continuously churn out the free-living cercarial stages that go on to infect the tuna.
The researchers then used specific sections of the DNA obtained from the parasites to match up the sac-like sporocyst stage in the worms with the adult stage in the tuna, and they were able to confirm that the blood flukes in the tuna were indeed originating from those infected tube-dwelling worms. As those sedentary worms usually live on the seafloor, researchers recommended that simply by moving them to deeper waters, the tuna would be infected by far fewer blood flukes. This study shows how understanding the ecology and life-cycle of a parasite can help us take straightforward measures that can mitigate their impact.
Photo by Robert Adlard
Reference:
Cribb TH, Adlard RD, Hayward CJ, Bott NJ, Ellis D, Evans D, Nowak BF. (2011) The life cycle of Cardicola forsteri (Trematoda: Aporocotylidae), a pathogen of ranched southern bluefin tuna, Thunnus maccoyii. International Journal for Parasitology 41:861-70.
August 30, 2011
Skrjabinoptera phrynosoma
Unlike most parasitic nematodes, which often lay eggs that are cast out of their host and left exposed to the elements, S. phrynosoma is a very maternal parasite - in a slightly morbid way. The female S. phrynosoma makes the ultimate sacrifice by casting her egg-filled body out of the lizard via the host's feces. She will die outside of the host - but in addition to protecting her eggs by doing so, it is also her strategy for helping her eggs reach the next host. For some reason, ants find the shriveled, egg-filled cadavers of female S. phrynosoma to be a tasty treat, a meal fit to feed to their brood of growing ant larvae - which then become infected with the parasite's own larvae. The life-cycle is complete when the infected larvae mature into workers, emerge from the colony, and become lizard food - horned lizards are specialists on ants.
Researchers at Georgia Southern University discovered that to ensure that this sequence of events occurs, S. phrynosoma has evolved to synchronise its life-cycle with the seasonal behaviour of both its lizard and ant hosts. They found that the number of egg-filled females (all ready to evacuate) reach peak abundance during the middle of the lizard's mating season. This is also the period when there are the greatest number of ants out busily foraging and when the colonies are packed to capacity with broods of growing ant larvae. By timing its life-cycle in such a manner, S. phrynosoma ensures that when next season rolls around, when those broods of larvae are ready to emerge as a new generation of workers ants, they will be doing so pre-infected with nematodes and just in time to welcome the hungry lizards coming out of hibernation.
Reference:
Hilsing, K.C., Anderson, R.A. and Nayduch, D. (2011) Seasonal dynamics of Skrjabinoptera phrynosoma (Nematoda) infection in horned lizards from the Alvord Basin: temporal components of a unique life-cycle. Journal of Parasitology 97: 559-564.
August 21, 2011
Caenorhabditis briggsae (KT0001)
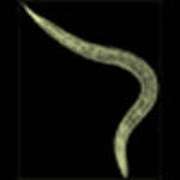
Worms in the genus Caenorhabditis are often associated with invertebrates, hitching a ride on them as a way of traveling between food sources, or even opportunistically feeding on their ride if it happens to drop dead for whatever reason. In a paper published last year, a group of researchers reported on a strain of C. briggsae (KT0001) from South Africa displaying an ability not previously known for any Caenorhabditis species - it is capable of infecting and killing wax moth larvae. This strain of C. briggsae was found to be in a symbiosis with the pathogenic bacteria Serratia which presumably allows C. briggsae (KT0001) to become a parasitic killer.
Furthermore, when the researchers tested 10 wild strains of Caenorhabditis species which had not previously displayed any ability to infect insects - including a strain of C. elegans - and cultured them with Serratia, all but one strain gained the ability to infect, kill, and reproduce in insects, including the famous C. elegans. It seems that Serratia gives Caenorhabditis a license to kill - upon forming a partnership with the bacteria, these worms turn from mere passengers into deadly killers.
Reference:
Abebe, E., Jumba, M., Bonner, K., Gray, V., Morris, K., Thomas, W.K. (2010) An entomopathogenic Caenorhabditis briggsae. Journal of Experimental Biology 213: 3223-3229.
August 10, 2011
Cytinus hypocistis
What makes C. hypocistis unusual is that while most fruit-bearing plants rely upon vertebrate animals to disperse their seeds, C. hypocistis mainly uses a beetle. Researchers found that the seeds collected from beetle frass (fancy name for insect poop) are just as viable as seeds which are collected directly from the fruit. While rodents and rabbits also frequently consume C. hypocistis fruits, because they have a tendency to eat immature fruits and deposit their dung (with any viable seeds) at ground level, they are not as effective as the beetles. Not only do the beetles consume only fully-ripened fruits, they also have a tendency to bury themselves into the sand during midday, which can bring the seeds closer to the roots of the host plant.
This is one of the few known case of endozoochory (where the seed is consumed and pass through the gut of an animal) which involves an insect. The researchers of this study pointed out that this type of ecological interaction may in fact be quite widespread and common, especially for plants with very small seeds. However, they have simply been overlooked because all those involved were, quite literally, lurking meekly underneath our feet.
Reference:
de Vega C, Arista M, Ortiz PL, Herrera CM, Talavera S (2011) Endozoochory by beetles: a novel seed dispersal mechanism. Annals of Botany 107: 629-637.
August 4, 2011
Isospora plectrophenaxia
Under natural condition, the usual light-dark cycle works just fine for most species of Isospora. But I. plectrophenaxia is found in the Snow Bunting (Plectrophenax nivalis) - a bird living in the High Arctic where there is perpetual sunlight during summer. So you'd think the shedding schedule of I. plectrophenaxia would be all messed up, right? Not so, researchers found that the parasite continues to stick to its regular regime of late afternoon shedding, just like all the other Isospora. At the moment researchers are unsure how I. plectrophenaxia is able to perform this feat. Perhaps this species is more sensitive to very low concentration of melatonin - the chemical secreted by the pineal organ which coordinates the bird's circadian rhythm, or perhaps it sets its timetable on different level of UV (ultraviolet) radiation exposure, which still varies throughout the Arctic summer day. Hopefully, ongoing research on this host-parasite system will shed further light on this little mystery, so watch this space!
Reference:
Dolnik O.V., Metzger B.J., Loonen M.J. (2011) Keeping the clock set under the midnight sun: diurnal periodicity and synchrony of avian Isospora parasites cycle in the High Arctic. Parasitology 138:1077-1081.
July 28, 2011
Acanthocephalus galaxii
Furthermore, the parasitic worm has actually become more abundant in the introduced trout than in the native galaxids - presumably because when compared with the tiny native fish, the much larger trout gobbles up more amphipods (the crustacean which carries the larval stage of A. galaxii). But this isn't necessarily good news for the parasite. Once they get into the trout, because of physiological incompatibility with the introduced host, the parasites are unable to reach maturity. So the trout actually acts as a kind of dead-end sink for the worm, which in turn reduces parasite burden on the native fishes.
So even while the trout might be chomping up native galaxids by the mouthful, they also are inadvertently reducing their parasite burden - though I doubt that would give much comfort to the little galaxids fleeing from a hungry trout!
References:
Paterson, R.A., Townsend, C.R., Poulin, R. and Tompkins, D.M. (2011) Introduced brown trout alter native acanthocephalan infections in native fish. Journal of Animal Ecology 88: 990-998.
July 18, 2011
Isospora lesouefi
Before the birds can be released into the wild, their health needs to be assessed and a part of that procedure involves determining their parasite load. For animals that you want to keep alive, this usually involves counting the number of parasite eggs or spores found in their faeces. But here's the tricky bit - it turns out that I. lesouefi keeps to a daily timetable. The researchers in this study found that bird faeces collected in the afternoon contained about 200 times more oocysts (the parasite's infective stage) than those collected in the morning. Other species of Isospora also keep similar shedding schedules, and it is likely to be an adaptive trait which minimise the oocysts' exposure to desiccation and ultraviolet radiation.
This study illustrates the importance of taking multiple samples, as well as understanding the life history of the parasites when you want to obtain an accurate picture of parasite burden, and its actual impact on the health of an animal.
Reference:
Morin-Adeline, V., Vogelnest, L. Dhand, N.K., Shiels, M., Angus, W. and Šlapeta, J. (2011) Afternoon shedding of a new species of Isospora (Apicomplexa) in the endangered Regent Honeyeater (Xanthomyza phrygia). Parasitology 138: 713-724
July 4, 2011
Myxidium sp.
The infamous cane toad does play a role in the story of Myxidium, but in a different manner to what was originally suspected. A collaborative group of researchers from Australia and the Czech Republic found that instead of bringing Myxidium to Australia, the toad has become embroiled in an ecological phenomenon known as "spillback". This is when a native parasite adopts a newly introduced host, this new species turns out to be a better host for the parasite than the native species it was originally infecting, and the parasite propogates more successfully in the new host species.
This can have dire consequences for the original host because the introduced species acts as an ampilifier for the parasite. As a result, the original host become exposed to more of the parasite than ever before. Because many parasites often have dose-dependent effects, this can mean a parasite, which would otherwise be tolerated, can become debilitating or even deadly to its original host.
Reference (and photo):
Hartigan A, Fiala I, Dyková I, Jirků M, Okimoto B, et al. (2011) A Suspected Parasite Spill-Back of Two Novel Myxidium spp. (Myxosporea) Causing Disease in Australian Endemic Frogs Found in the Invasive Cane Toad. PLoS ONE 6(4): e18871. doi:10.1371/journal.pone.0018871
June 14, 2011
Trypanosoma irwini
In addition to T. irwini, the Koala is also infected by two other species of Trypanosoma. While on its own, T. irwini seems to be pretty benign, if it gets mixed up with the other Trypanosoma species or other infections such as chlamydia or the retrovirus which causes koala AIDS syndrome, it can lead to disease in its host. Like many other parasites, the pathogenecity of T. irwini is not so straightforward, and may only manifest itself under certain conditions.
Photo from McInnes et al. (2009)
References:
McInnes, L.M., Gillett, A., Ryan, U.M., Austen, J., Campbell, R.S.F., Hanger, J. and Reid, S.A. (2009) Tryapnosoma irwini n. sp. (Sarcomastigophora: Trypanosomatidae) from the koala (Phascolarctos cinereus). Parasitology 136: 875-885.
McInnes, L.M., Gillett, A.,Hanger, J., Reid, S.A. and Ryan, U.M. (2011) The potential impact of native Australian trypanosome infections on the health of koala (Phascolarctos cinereus). Parasitology 138: 873-883
June 4, 2011
Gnathia auresmaculosa
Settlement is a critical transitional stage for coral reef fishes, and that is also when they are most vulnerable to parasites like G. auresmaculosa. A recent study by the lab group of Dr. Alexandra Grutter revealed just how costly these ticks of the sea can be to juvenile fishes. Dr. Grutter and her colleagues found that juvenile damselfish which have been fed on by just one of those little blood-suckers exhibit significantly decreased swimming ability, far higher oxygen consumption rate, and are about half as likely to survive than uninfected fishes.
So if you happen to find yourself on a beautiful tropical reef, take a moment to think about all the little baby fishes which are swimming for their lives through the gauntlet of gnathiids - they never mentioned that in Finding Nemo!
Reference:
Grutter, A.S., Crean, A.J., Curtis, L.M., Kuris, A.M., Warner, R.R. and McCormick, M.I. (2011) Indirect effects of an ectoparasite reduce successful establishment of a damselfish at settlement. Functional Ecology 25: 586-594
May 29, 2011
Eustrongylides ignotus
Contributed by Mike Kinsella.
May 18, 2011
Clistobothrium carcharodoni
Tapeworms in general have complex life-cycles with many different hosts, and C. carcharodoni is no different. The life cycle of tapeworms which live in marine animals such as the great white shark are difficult to unravel. That is because the larvae lack many of the diagnostic characteristics which are used to identify the adult worms, so it is next to impossible to match the identity of the larvae with adults based on their morphologies. But with the advent of molecular techniques such mystery are becoming more commonly solved.
One of my former colleagues from Otago University - Haseeb Randhawa - was able to use key genetic markers to confirm that adult C. carcharodoni found in the gut of great white sharks were identical to tapeworm larvae which have previously been found in dolphins. These larval tapeworms congregate in the tail, back, belly and groin region of the dolphins - all parts preferred by the great white sharks as the finest cuts of meat from Flipper. His study confirmed the role of dolphins in completing the life-cycle of C. carcharodoni.
So while Flipper and Jaws are famous superstars which grab all the public attention, to a tapeworm like C. carcharodoni, all those aquatic celebrities simply serve as way stations in the cycle of life.
Reference:
Randhawa, H. (2011) Insights using a molecular approach into the life cycle of a tapeworm infecting great white sharks. Journal of Parasitology 97: 275-280.
May 15, 2011
Chondracanthus parvus
Picture from Ho et al. (2006).
References:
Paterson, A.M. and Poulin, R. (1999) Have chondracanthid copepods co-speciated with their teleost hosts? Systematic Parasitology 44:79-85.
Ho, J-s., Kim, I-H., and Nagasawa, K. (2006) Copepod parasites of the fatheads (Pisces, Psychrolutidae) and their implication on the phylogenetic relationships of Psychrolutid genera. Zoological Science 22:411-425.
April 17, 2011
Herpyllobius vanhoeffeni
The top picture shows a pair of females attached to the ventral surface of their host; note that the lower individual has a pair of lobe-shaped egg sacs extending from its side like wings. The bottom picture shows a specimen that has been dissected from the host, showing the rest of the copepod, which is usually embedded in the host. Overall, the whole parasite looks not unlike a bulbous skull resting atop a twisted stalk of a body.
Reference:
López-González, P.J. and Bresciani, J. (2001) New Antarctic records of Herpyllobius Steenstrup and Lütken, 1861 (parasitic Copepoda) from the EASIZ-III cruise, with description of two new species. Scientia Marina 65:357-366
April 9, 2011
Columbicola extinctus
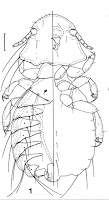
April 7, 2011
Ixodes neuquenensis
Today's parasite is a tick described from an endangered marsupial. Ixodes neuquenensis is an ectoparasite of a unique little marsupial known as monito del monte or "mountain monkey" (Dromiciops gliroides).
The "mountain monkey" is the only species still alive from an ancient lineage dating back more than 40 million years. Due to habitat loss, the population of this little marsupial has declined over recent years. This is bad news for I. neuquenensis because it is a very host-specific tick. If the "mountain monkey" goes extinct, it will also spell doom for this tick, along with a whole suite of other parasites and symbionts which are dependent upon this little marsupial.
Reference:
Guglielmone AA, Venzal JM, Amico G, Mangold AJ, Keirans JE (2004) Description of the nymph and larva and redescriptions of the female of Ixodes neuquenensis Ringuelet, 1947 (Acari: Ixodidae), a parasite of the endangered Neotropical marsupial Dromiciops gliroides Thomas (Microbiotheria: Microbiotheriidae). Systematic Parasitology 57:211–219
April 2, 2011
Philophthalmus gralli - update on the "Parasite of 2010"
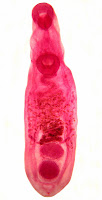
Contributed by Mike Kinsella.
March 23, 2011
Rugogaster hydrolagi
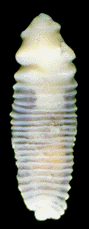
Photograph by Klaus Rohde
March 17, 2011
Pygidiopsis macrostomum
Pygidiopsis macrostomum is a freshwater digenean from Brazil. Like most otherdigenean trematodes, it has a three-host life-cycle. It asexually multiplies in its first intermediate host, the snail Heleobia australis, producing cercariae (pictured) which are released into the surrounding water. The cercaria swims in an series of small, stepped leaps, and then spins rapidly on its own axis once it sinks to the substrate, almost like a tiny aquatic ballerina.
All this dance-like motion attracts the attention of guppies, the parasite's second intermediate hosts, which ingest the parasite and become infected. The parasites burrow into the mesentery tissue of the fish, where they form a cyst and await ingestion by the definitive host where the worm will mature into its adult stage. While the adult specimen of P. macrostomum were first described from a rat, a subsequent study have also found it in the piscivorous bat Noctilio leporinus which, given its diet, is more likely to be the parasite's usual definitive host.
Reference:
Simões et al. (2009) The life history of Pygidiopsis macrostomum Travassos, 1928 (Digenea: Heterophyidae). Mem Inst Oswaldo Cruz 104:106-111.
Contributed by Tommy Leung.
February 20, 2011
Opechona sp.
References:
Averbuj, A. and Cremonte, F. (2010) Parasitic castration of Buccinanops cochlidium (Gastropoda: Nassariidae) caused by a lepocreadiid digenean in San José Gulf, Argentina. Journal of Helminthology 84: 381–389.
Contributed by Tommy Leung.
February 5, 2011
Colobomatus sillaginis
Reference:
West, G.A. (1983) A new philichthyid copepod parasitic in whiting (Sillago spp.) from Australian waters. Journal of Crustacean Biology 3: 622-628.
Contributed by Tommy Leung.
January 30, 2011
Allomermis solenopsi
Image from figure of the paper.
Reference:
Poinar Jr, G.O., Porter, S.D., Tang, S. and Hyman, B.C. (2007) Allomermis solenopsi n. sp. (Nematoda: Mermithidae) parasitising the fire ant Solenopsis invicta Buren (Hymenoptera: Formicidae) in Argentina. Systematic Parasitology 68: 115-128.
Contributed by Tommy Leung.
Reference:
Poinar Jr, G.O., Porter, S.D., Tang, S. and Hyman, B.C. (2007) Allomermis solenopsi n. sp. (Nematoda: Mermithidae) parasitising the fire ant Solenopsis invicta Buren (Hymenoptera: Formicidae) in Argentina. Systematic Parasitology 68: 115-128.
Contributed by Tommy Leung.
January 24, 2011
Nearctopsylla brooksi
Contributed by Mike Kinsella.
Subscribe to:
Posts (Atom)